When you hear about alien lifeforms, one of the most common phrases is "silicon-based life." The idea crops up often in science fiction—but what does it mean? Would it really look like burnt pizza? Is it even possible? What other forms of life are there?
Previously, we looked at the basic concepts of evolution from the perspective of research biology. If you haven't already read Part I, you might wish to do so.
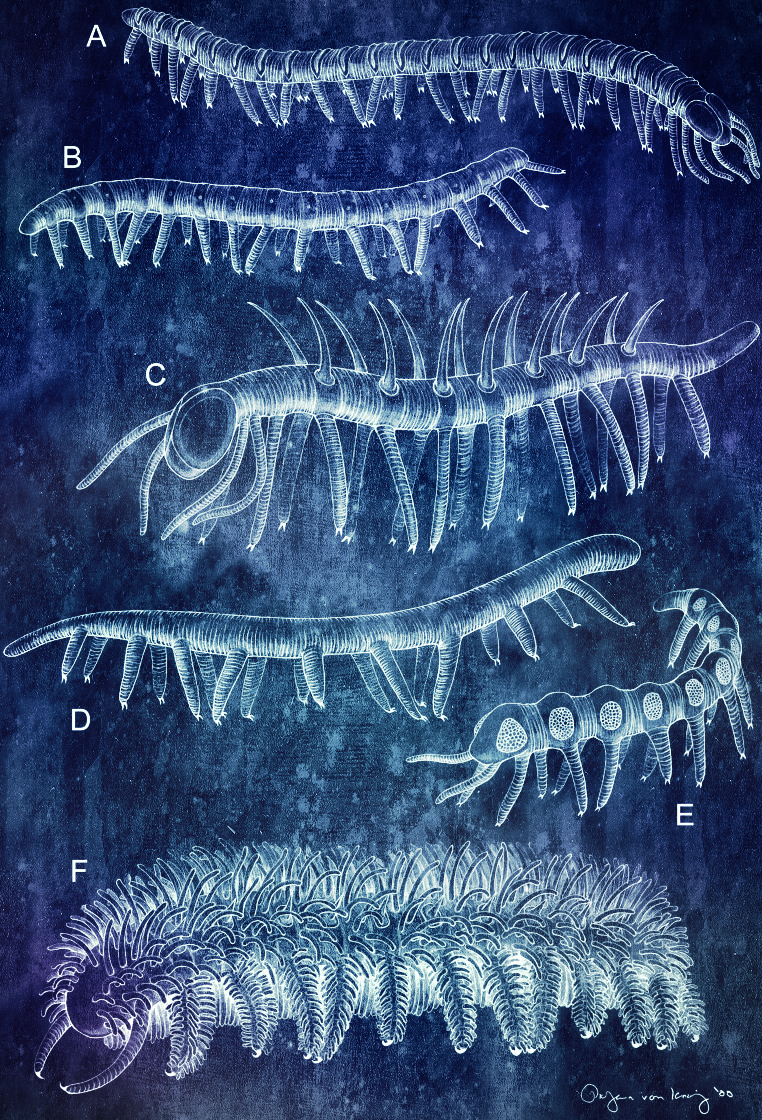
The question of what is permissible in the formation life has been of interest to biologists ever since we first started noticing that certain arbitrary decisions have occurred—the most obvious of which ischirality, i.e. that certain molecules have mirrored versions, and we only use one set. This is the most basic form of alternative biochemistry, and particularly convenient because we can be confident that flipped chirality would be a viable form of life. The chirality we have in our own biomolecules most likely originates from random chance.
However, a direct transposition of chirality with nothing else changed about the biological makeup of an alien lifeform would be rather exceptional. Many of the strategies evolved by life on Earth are peculiar to the abundances of natural and artificial (i.e., life-generated) resources, and even then, there are many important yet arbitrary decisions, like the codon table, which is the key the cell uses to convert nucleotide triplets into amino acids during protein production. Most likely, any successful chemically-based life form will preserve the following few traits:
Some kind of information storage molecule that acts as a permanent record. This storage molecule must be capable of mutating, easy to read from, and relatively stable. We use DNA, which is great if you're a carbon-and-water—based lifeform that exists predominantly at temperatures below 80° C. The double-stranded structure is particularly useful for data redundancy, but it's easy to chop up and alter if needed. Many viruses use RNA or a single-stranded version of DNA. A separate information storage molecule is critical to permit evolution. While there are a small number of cases known where proteins propagate alterations to each other directly (prions), this mechanism is very decentralized, and evolution appears to have tried to avoid it.
Some kind of functional molecule that can alter the world around it, catalyse chemical reactions, and ideally interact with the information storage mechanism. These molecules must be derivable from the information storage molecules in some way (ideally by copying), they must have three-dimensional structure, and they must have a wide range of ways in which they can affect their environments. We use proteins, and to a lesser extent RNA can also perform a limited range of functions (which are called ribozymes).
Some way to compartmentalize reactions to protect these molecules from each other, to store raw materials for later, and to protect all of the above from the outside world. We use phospholipids (a kind of fat), which are particularly useful because they block the flow of water, and membranes made out of phospholipids grow and shrink easily when more are inserted or removed.
It's worth noting that there is a great deal of evidence that DNA and proteins were both added later—life on Earth may have originally used the more delicate RNA instead of DNA.
But back to our first question. What can we change about the chemistry itself?
Silicon-based life has some problems. It takes more energy to form and break bonds with silicon than with carbon, and when silicon does form bonds with certain useful non-metals like oxygen, it tends to result in long polymer repeats, not irregular short structures, and is highly reactive when it bonds to itself. While silicon almost certainly has enough versatility to function in a complex living organism as a replacement for carbon in some or all positions, it is much worse from the perspective of evolution. As a result, life is inherently much less likely to evolve using silicon than carbon. (If any environment is a haven for silicon life, though, it'd be a strongly acidic or high-pressure one.)
Scientists have also looked at using boron, sulphur, and a mixture of nitrogen and phosphorus as alternatives to carbon. For the most part, these are very reactive and volatile, although boron may be more useful in an ammonia atmosphere—if it's abundant enough, which it generally isn't.
In fact, there are a lot of other elements that might do a job reasonably well, but are widely believed to be too rare in the universe to have a chance: chlorine could replace oxygen, selenium could replace sulphur, and hydrogen fluoride could replace water. Many of these are hilariously toxic to life on Earth—especially hydrogen fluoride, which can eat through glass. But there are proposals for combinations of alternatives that would complement each other—to wit, hydrogen fluoride doesn't react with paraffin (candle wax).
Conversely to the problem of 'too rare', the idea of life without abundant hydrogen (the most common element in the universe) has been proposed, which would use oxygen or ionic bonds with metals to pad carbon chains instead. Normally, hydrogen is an extremely useful element to life because it means not every bond in a carbon molecule has to be put to use doing something useful; it can simply be ignored by sticking on a free proton. The prodigious abundance of hydrogen in the universe (91% of all atoms by count; 71% of all atomic matter by mass) makes this sort of thinking very peculiar.
The idea that certain elements or chemicals must perform the role they do in our world for life to be possible is called chauvinism of that chemical; e.g. carbon chauvinism or water chauvinism, terms coined by the astronomer Carl Sagan. Carbon chauvinism is relatively easy to justify on account of the extreme rarity of the alternatives, but the second most essential chemical anthropocentrism, water chauvinism, is not generally insisted upon. Ammonia is a very scientifically plausible alternative—it can donate and accept protons just like water, it is liquid at roughly the same temperatures (up to a point, anyway—it boils at a lower temperature), and it is polar. Nitrogen is also very abundant, although not as abundant as oxygen.
Many conciliatory changes would be required to accommodate ammonia-based life; most of the uses of oxygens in biological molecules would need to be replaced with nitrogen, for example, and the atmosphere itself would have to contain much less oxygen than ours does, which would make it reducing rather than oxidizing. Incidentally, ours once did—until early plants messed it up. This was called the Great Oxygenation Event.
There are also some lingering problems with using ammonia in this way—splitting oxygen is a very accessible energy source, and performing the same with nitrogen isn't. This is why the Great Oxygenation Event happened; the development of an oxygen-rich atmosphere made growth easier. In addition, ammonia is notably less polar than water, which means it is less effective at holding together hydrophobic protein cores and phospholipid bilayer membranes. Alternatives to these may need to be found for life to be really successful.
This all being said, though, it's possible that not all life in the universe is chemical, at least not in the traditional sense. Transposons are a special kind of gene that move around on the chromosome spontaneously. They include instructions for making special cut-and-paste proteins that allow them to do this, and the cell's normal machinery unwittingly moves them around in response. To a computer scientist, it is easy to see how such a system could be made Turing-complete, i.e. capable of being manipulated so it can calculate any mathematical or logical function that a computer can solve.
Transposons share a lot in common with viruses, in that while they are not independent organisms capable of maintaining homeostasis (internal chemical balance) and truly reacting to their environment, they both hijack the cell's own processes to replicate and transmit themselves. Most biologists do not consider either to constitute life, but who knows—if they evolve far enough to start demanding civil rights, we might not have to complain.
In Part III, we'll look at the possibility that they already have—and some other neat things about Earth's most financially successful bipeds.
Previously, we looked at the basic concepts of evolution from the perspective of research biology. If you haven't already read Part I, you might wish to do so.
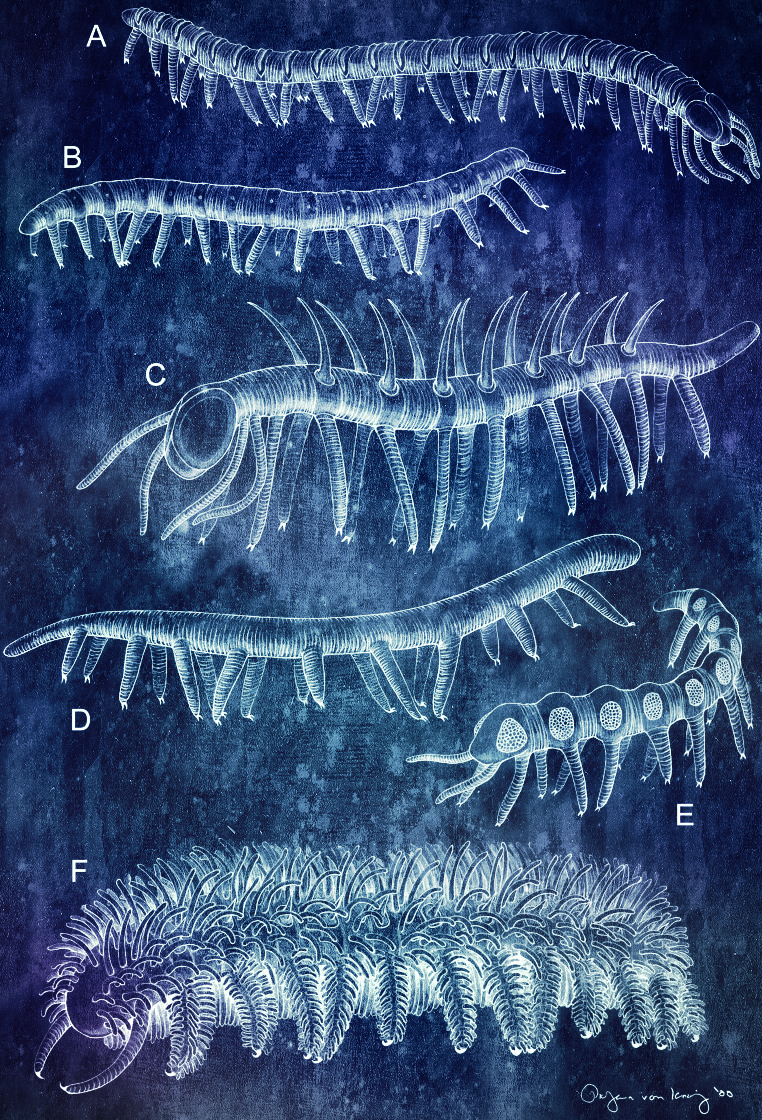
Examples from the phylum Onychophora, one of the most peculiar kinds of worms.
The question of what is permissible in the formation life has been of interest to biologists ever since we first started noticing that certain arbitrary decisions have occurred—the most obvious of which ischirality, i.e. that certain molecules have mirrored versions, and we only use one set. This is the most basic form of alternative biochemistry, and particularly convenient because we can be confident that flipped chirality would be a viable form of life. The chirality we have in our own biomolecules most likely originates from random chance.
However, a direct transposition of chirality with nothing else changed about the biological makeup of an alien lifeform would be rather exceptional. Many of the strategies evolved by life on Earth are peculiar to the abundances of natural and artificial (i.e., life-generated) resources, and even then, there are many important yet arbitrary decisions, like the codon table, which is the key the cell uses to convert nucleotide triplets into amino acids during protein production. Most likely, any successful chemically-based life form will preserve the following few traits:
It's worth noting that there is a great deal of evidence that DNA and proteins were both added later—life on Earth may have originally used the more delicate RNA instead of DNA.
But back to our first question. What can we change about the chemistry itself?
Silicon-based life has some problems. It takes more energy to form and break bonds with silicon than with carbon, and when silicon does form bonds with certain useful non-metals like oxygen, it tends to result in long polymer repeats, not irregular short structures, and is highly reactive when it bonds to itself. While silicon almost certainly has enough versatility to function in a complex living organism as a replacement for carbon in some or all positions, it is much worse from the perspective of evolution. As a result, life is inherently much less likely to evolve using silicon than carbon. (If any environment is a haven for silicon life, though, it'd be a strongly acidic or high-pressure one.)
Scientists have also looked at using boron, sulphur, and a mixture of nitrogen and phosphorus as alternatives to carbon. For the most part, these are very reactive and volatile, although boron may be more useful in an ammonia atmosphere—if it's abundant enough, which it generally isn't.
In fact, there are a lot of other elements that might do a job reasonably well, but are widely believed to be too rare in the universe to have a chance: chlorine could replace oxygen, selenium could replace sulphur, and hydrogen fluoride could replace water. Many of these are hilariously toxic to life on Earth—especially hydrogen fluoride, which can eat through glass. But there are proposals for combinations of alternatives that would complement each other—to wit, hydrogen fluoride doesn't react with paraffin (candle wax).
Conversely to the problem of 'too rare', the idea of life without abundant hydrogen (the most common element in the universe) has been proposed, which would use oxygen or ionic bonds with metals to pad carbon chains instead. Normally, hydrogen is an extremely useful element to life because it means not every bond in a carbon molecule has to be put to use doing something useful; it can simply be ignored by sticking on a free proton. The prodigious abundance of hydrogen in the universe (91% of all atoms by count; 71% of all atomic matter by mass) makes this sort of thinking very peculiar.
The idea that certain elements or chemicals must perform the role they do in our world for life to be possible is called chauvinism of that chemical; e.g. carbon chauvinism or water chauvinism, terms coined by the astronomer Carl Sagan. Carbon chauvinism is relatively easy to justify on account of the extreme rarity of the alternatives, but the second most essential chemical anthropocentrism, water chauvinism, is not generally insisted upon. Ammonia is a very scientifically plausible alternative—it can donate and accept protons just like water, it is liquid at roughly the same temperatures (up to a point, anyway—it boils at a lower temperature), and it is polar. Nitrogen is also very abundant, although not as abundant as oxygen.
Many conciliatory changes would be required to accommodate ammonia-based life; most of the uses of oxygens in biological molecules would need to be replaced with nitrogen, for example, and the atmosphere itself would have to contain much less oxygen than ours does, which would make it reducing rather than oxidizing. Incidentally, ours once did—until early plants messed it up. This was called the Great Oxygenation Event.
There are also some lingering problems with using ammonia in this way—splitting oxygen is a very accessible energy source, and performing the same with nitrogen isn't. This is why the Great Oxygenation Event happened; the development of an oxygen-rich atmosphere made growth easier. In addition, ammonia is notably less polar than water, which means it is less effective at holding together hydrophobic protein cores and phospholipid bilayer membranes. Alternatives to these may need to be found for life to be really successful.
This all being said, though, it's possible that not all life in the universe is chemical, at least not in the traditional sense. Transposons are a special kind of gene that move around on the chromosome spontaneously. They include instructions for making special cut-and-paste proteins that allow them to do this, and the cell's normal machinery unwittingly moves them around in response. To a computer scientist, it is easy to see how such a system could be made Turing-complete, i.e. capable of being manipulated so it can calculate any mathematical or logical function that a computer can solve.
Transposons share a lot in common with viruses, in that while they are not independent organisms capable of maintaining homeostasis (internal chemical balance) and truly reacting to their environment, they both hijack the cell's own processes to replicate and transmit themselves. Most biologists do not consider either to constitute life, but who knows—if they evolve far enough to start demanding civil rights, we might not have to complain.
In Part III, we'll look at the possibility that they already have—and some other neat things about Earth's most financially successful bipeds.